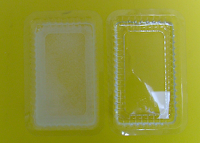 |
Figure 1. Two shallow plastic containers. The left one holds a lot of salt and the right one is plain water. A small amount of water was added to the left one. |
This is the fourth followup of the blog article: "
A perfect storm in a cup of salt water?" that started this journey of discovery.
The vapor pressure lowering is an effect that says the water vapor pressure above saltwater is lower than that above freshwater. This is more generally described by Raoult's Law, which states that the vapor pressure of an ideal solution depends on the vapor pressure of each chemical component and the mole fraction of the component present in the solution. Since the sodium and chlorine ions hardly evaporate, the vapor pressure above saltwater comes from the evaporation of water molecules.
The molecular mechanism behind the vapor pressure lowering is easy to understand--the ions stay in the way of water molecules and slow down the rate of their evaporation and, in the case of salt, they even act to attract the water molecules and prevent them from leaving the solution.
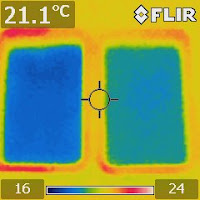 |
Figure 2. An IR image of the two shallow containers right after water was added to the salt one on the left. |
Let's try to use infrared (IR) imaging to visualize this process. Prepare two plastic containers like the ones shown in Figure 1. Add plenty of salt to one of them and some water to the other. Then add some water to the salt one. Figure 2 shows an IR image just after water was added. The image shows that the system absorbed heat while salt was being dissolved.
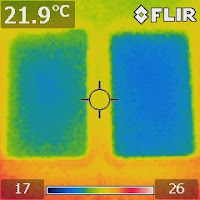 |
Figure 3. An IR image after half an hour
showing that the evaporative cooling
effect of the saltwater container is weaker
than the pure water one. |
Let the containers sit for about half an hour and then take another IR image. Figure 3 shows the result. Interestingly, the colors reversed. Now, the saltwater container appears to be warmer than the pure water one.
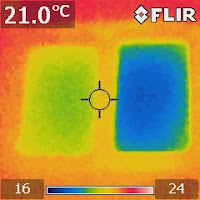 |
Figure 4. An IR image after a few hours
showing that the contrast of colors
became greater. |
Wait for a few hours and then come back to take an IR shot. Figure 4 shows that the temperature difference became greater.
How to interpret these results? There are two mechanisms that cause the temperature difference.
One is the vapor pressure lowering mentioned above. Using a
Vernier relative humidity sensor, one can confirm that the humidity above the saltwater is lower than that above the freshwater. This means that the evaporation weakens above saltwater, which reduces the cooling effect.
The other is the crystallization of salt that releases heat. The evaporation of every water molecule weakens the ability of the solution to hold ions. As water constantly evaporates, a corresponding amount of ions must return to the crystalline form--mostly at the bottom because the contact area with the wall is much smaller compared with the contact area with the bottom. This process releases heat at the bottom. Since the saltwater is very shallow, the heat conduction may happen fast enough so that the crystallization heat will pass to the surface of the saltwater--even if convection may be insignificant with such a shallowness--and make it even warmer on top of the weaker evaporative cooling effect. This effect, which is totally based on molecular reasoning, is yet to be confirmed by an experimental method.
The vapor pressure lowering process and the crystallization process in this system are intertwined. If evaporation slows down (absorb less heat) due to salt, crystallization slows down (release less heat) too. The small amount of crystallization heat transfers to the surface and slightly increases the evaporation rate, which in turn causes slightly more ions to crystallize. The two processes manage to keep the saltwater container warmer than the freshwater container. But we still don't know which process contributes more. The question is, without the crystallization heat, can the IR image of the saltwater be as warm as it appears to be? How can we separate the two effects? Sealing the containers to stop evaporation doesn't work because that will stop crystallization as well.
Why do I insist on the theory of crystallization heat? Not only because it is logical. If we look at Figure 2, we will see that the effect of heat of solution is pretty significant. In order for salt to dissolve in water, some heat needs to be absorbed. Now, when the reverse process has to happen, i.e., when the salt ions have to return to the solid form, the same amount of heat must be released--in a much slower pace because of the low rate of evaporation (compared with the rate of dissolving). This is just simply the rule of energy conservation at work. The chemical potential must act like a spring. Energy is stored when it is "compressed" and released when it "bounces back."
Most likely, I now think this mysterious effect in a cup of saltwater is an orchestration of many physical and chemical effects. The salt gradient in a saturated solution is yet another mystery to be uncovered: the salinity gradient exists only in a saturated solution but not in any unsaturated solution.
A small cup of saltwater may contain a lot of physical chemistry! Stay tuned for more follow-up experiments.