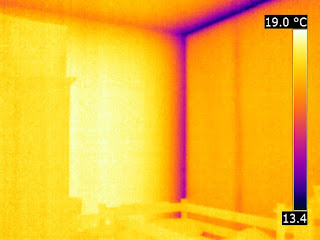 |
Fig. 1: IR image of a wall junction (inside) by Stefan Mayer |
One of the mysterious things that causes people to scratch their heads when they see an infrared picture of a room is that the junctions such as edges and corners formed by two exterior walls (or floors and roofs) often appear to be colder in the winter than other parts of the walls, as is shown in Figure 1. This is, I hear you saying, caused by an air gap between two walls. But not that simple! While a leaking gap can certainly do it, the effect is there even without a gap. Better insulation only makes the junctions less cold.
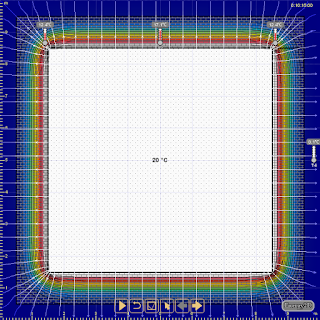 |
Fig. 2: An Energy2D simulation of thermal bridge corners. |
A typical explanation of this phenomenon is that, because the exterior surface of a junction (where the heat is lost to the outside) is greater than its interior surface (where the heat is gained from the inside), the junction ends up losing thermal energy in the winter more quickly than a straight part of the walls, causing it to be colder. The temperature difference is immediately revealed by a very sensitive IR camera. Such a junction is commonly called a
geometric thermal bridge, which is different from
material thermal bridge that is caused by the presence of a more conductive piece in a building assembly such as a steel stud in a wall or a concrete floor of a balcony.
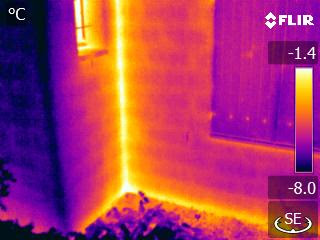 |
Fig. 3: IR image of a wall junction (outside) by Stefan Mayer |
But the actual heat transfer process is much more complicated and confusing. While a wall junction does create a difference in the surface areas of the interior and exterior of the wall, it also forms a thicker area through which the heat must flow through (the area is thicker because it is in a diagonal direction). The increased thickness should impede the heat flow, right?
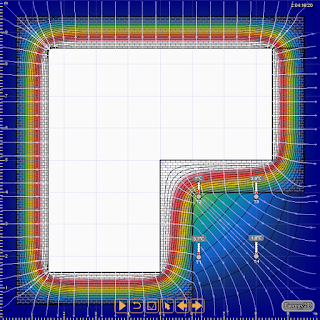 |
Fig. 4: An Energy2D simulation of a L-shaped wall. |
Unclear about the outcome of these competing factors, I made some
Energy2D simulations to see if they can help me. Figure 2 shows the first one that uses a block of object remaining at 20
°C to mimic a warm room and the surrounding environment of 0
°C, with a four-side wall in-between. Temperature sensors are placed at corners, as well as the middle point of a wall. The results show that the corners are indeed colder than other parts of the walls in a stable state. (Note that this simulation only involves heat diffusion, but adding radiation heat transfer should yield similar results.)
What about more complex shapes like an L-shaped wall that has both convex and concave junctions? Figure 3 shows the IR image of such a wall junction, taken from the outside of a house. In this image, interestingly enough, the convex edge appears to be colder, but the concave edge appears to be warmer!
The Energy2D simulation (Figure 4) shows a similar pattern like the IR image (Figure 3). The simulation results show that the temperature sensor placed near the concave edge outside the L-shape room does register a higher temperature than other sensors.
Now, the interesting question is, does the room lose more energy through a concave junction or a convex one? If we look at the IR image of the interior taken
inside the house (Figure 1), we would probably say that the convex junction loses more energy. But if we look at the IR image of the exterior taken
outside the house (Figure 3), we would probably say that the concave junction loses more energy.
Which statement is correct? I will leave that to you. You can download the Energy2D simulations from this
link, play with them, and see if they help you figure out the answer. These simulations also include simulations of the reverse cases in which heat flows from the outside into the room (the summer condition).