In the year 2014, Energy2D
has incorporated a radiation simulation engine and a particle simulation
engine, expanding its modeling capacity and making it a truly multiphysics
simulation package. To celebrate the New Year, I made some simulations
that demonstrate these multiphysics features using objects shaped after the
numbers of 2015.
These simulations feature the fluid dynamics engine, the heat conduction engine, the thermal radiation engine, and the particle dynamics engine. If you are curious enough, you can click this link to run the simulations.
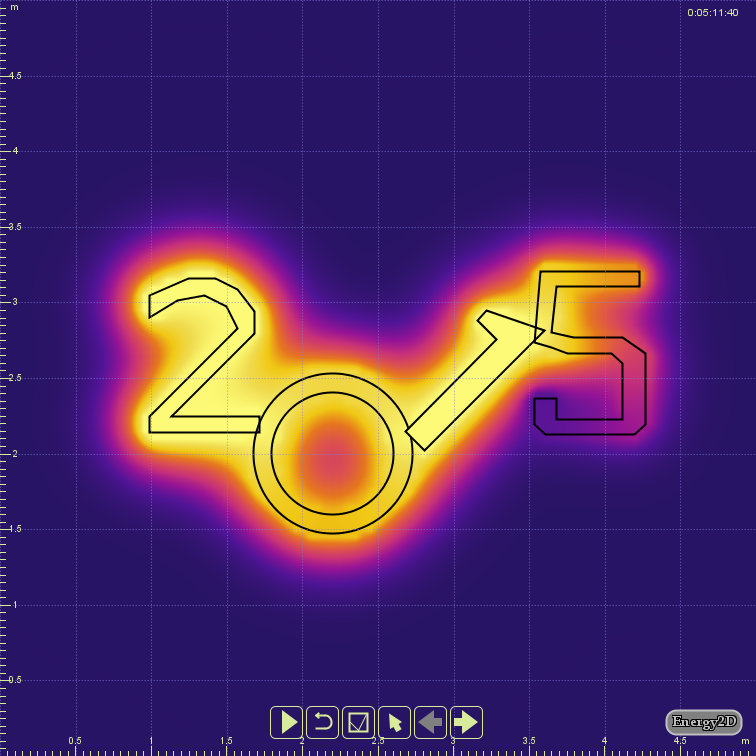
These shapes were drawn using Energy2D's polygon and ring tools, which allow users to create a wide variety of arbitrary 2D shapes.
Many users probably do not know how versatile the polygon tool actually is (the original triangle icon on the tool bar probably misleads some to think it is only good for drawing triangles -- so I changed it to look like a cross-section of an I-beam). The polygon tool
allows one to easily draw a polygon with maximally 256 control points for adjusting its shape later. One can draw an approximate shape and then drag these control points to get it to the exact shape. To modify a shape even further, one can also insert a control point by double-clicking on an existing point. A new point will be added to the adjacent position, which you can then drag around. To delete a control point, just hold down the SHIFT key while double-clicking on it. In addition, a polygon can be rotated, twisted, compressed, or elongated using the corresponding fields in its property window (there is currently no graphical user interface for doing those things, however).
As for the New Year's resolutions, in 2015, the ring shape will be enhanced into a new tool called the shape subtractor, which allows users to subtract a shape from another to make a hollow one.
On the numerical simulation side, we will continue to improve the accuracy of the existing simulation engines by adding an explicit solver as an option for users to overcome some of the problems related to the implicit solvers.
On the multiphysics modeling side, we will try to support multiple fluids, which seems simple at first glance but has turned out to be a very difficult mathematical problem. With the capacity of multiple fluids, we will also be able to add an electromagnetism solver in order to model effects such as electrorheological fluids (fluids whose viscosity changes with respect to an applied electric field).
We wish all Energy2D users a very successful new year!
These simulations feature the fluid dynamics engine, the heat conduction engine, the thermal radiation engine, and the particle dynamics engine. If you are curious enough, you can click this link to run the simulations.
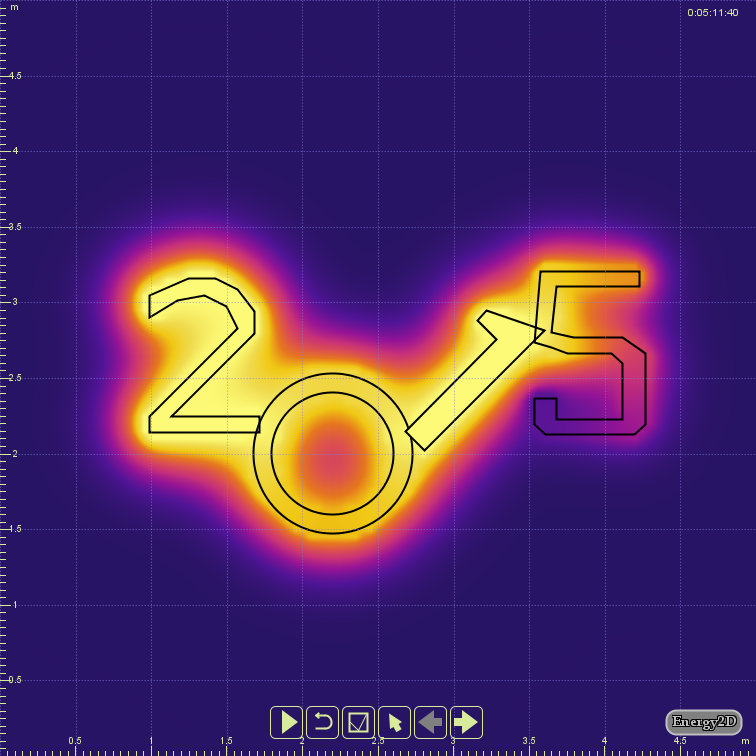
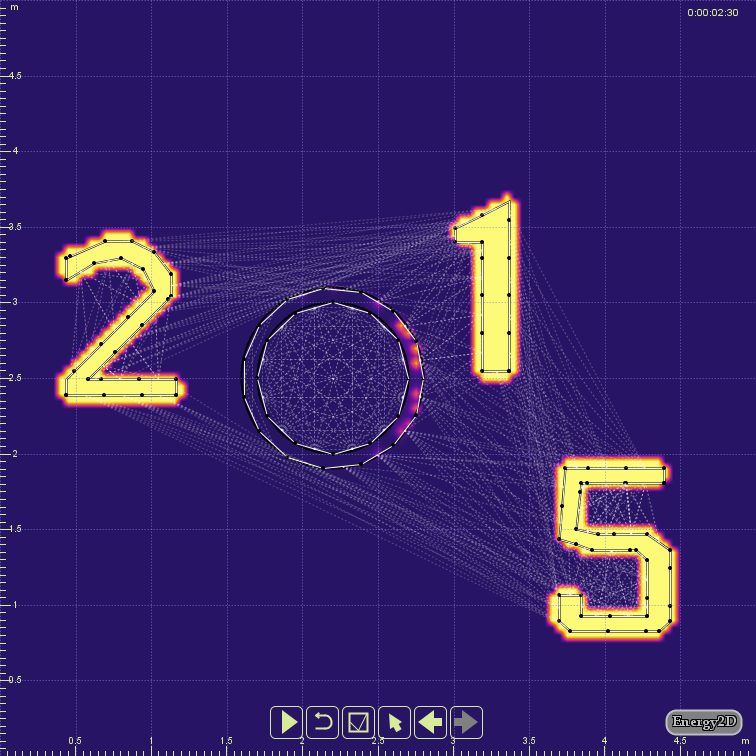
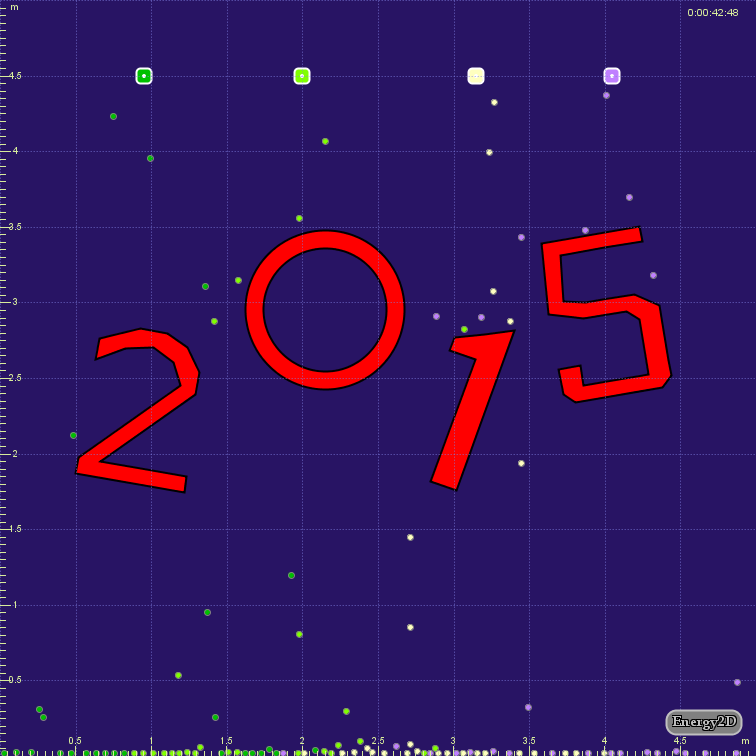
On the numerical simulation side, we will continue to improve the accuracy of the existing simulation engines by adding an explicit solver as an option for users to overcome some of the problems related to the implicit solvers.
On the multiphysics modeling side, we will try to support multiple fluids, which seems simple at first glance but has turned out to be a very difficult mathematical problem. With the capacity of multiple fluids, we will also be able to add an electromagnetism solver in order to model effects such as electrorheological fluids (fluids whose viscosity changes with respect to an applied electric field).
We wish all Energy2D users a very successful new year!